В БАК впервые произошли столкновения протонов на рекордной энергии 7 ТэВ
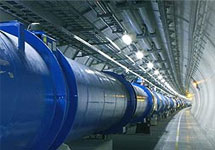
Туннель ускорителя большого адронного коллайдера. Фото с сайта CERN
Физики Европейской организации ядерных исследований (ЦЕРН) впервые успешно осуществили столкновения протонов в Большом адронном коллайдере на рекордной энергии 7 тераэлектрон-вольт, что в три раза больше прежнего рекорда.
Комментарии
hmm
hmhm
http://cdsweb.cern.ch/journal/CERNBulletin/2010/31/News%20Articles/1281662http://indico.cern.ch/getFile.py/access?contribId=73&sessionId=13&resId=1&materialId=slides&confId=73513 http://www.ichep2010.fr/ And so let's do everything in this way. After all, it will be better. I mean explained this message by references : http://www-d0.fnal.gov/Run2Physics/D0ICHEP2010.html http://www-d0.fnal.gov/Run2Physics/D0abstractsSUSY2010.pdf http://www.ichep2012.com.au/http://indico.cern.ch/getFile.py/access?contribId=73&sessionId=13&resId=1&materialId=slides&confId=73513 And one more thing that can be explained even remotely, and in general : -New results are as follows: the Higgs boson is not visible, but the range of masses, in which the existence of boson "closed" on the confidence level of 95%, increased up to 158-175 GeV. Here, a small and somewhat no detailed explanation: Therefore, according to the data gathered at the LEP collider at CERN turned out that an experimental lower bound to be set for the mass of the Standard Model Higgs boson of 114.4 GeV at 95% confidence level. But before it was considered that: - the Higgs mass could be kept small only by a delicate fine-tuning of parameters(and only). At the Fermilab Tevatron, there are ongoing experiments searching for the Higgs boson,and in July 2010, combined data from CDF and D0experiments at the Tevatron were sufficient to exclude the Higgs boson in the range between 158 GeV and 175 GeV at the 95% confidence level.
A heavy Higgs ( 2mZ < mH < 650 GeV)
Well assumed so-called the golden channel for Higgs decays:That if a standard model Higgs will be having a mass above twice the Z mass the discovery will be easy through the decay channel H ZZ l +l -l +l -. .A heavy Higgs (2mZ
Strictly speaking, nothing has changed. Or rather no surprises are expected in the search for the Higgs boson (yet). But it should be noted that the several alternatives to the Higgs mechanism have been proposed and all of the alternative mechanisms use strongly interacting dynamics to produce a vacuum expectation value that breaks electroweak symmetry. One of this mechanisms is techniclor. This mechanism is a class of models that attempts to mimic the dynamics of the strong force as a way of breaking electroweak symmetry (roughly speaking)... The Higgs lagrangian is replaced by suitable special fermion sector interacting strongly via fifth force (technicolor) .Lhiggs .--.>(-1/4) Fmunu F^munu + iQ_ymu d^mu Q . Well, it was assumed that if this could be realized, then the chiral -flavor symmetries would be broken spontaneously as for ordinary QCD . Classical technicolor is heavily inspired by QCD. It was invented at a time when the heaviest the fermions was the bottom quark it was much less than the weak scales. For QCD- like technicolor this scale is ~3 TeV, while for a walking theory with d=2 it is ~10TeV.This means that in this framework the dynamics that generates the top quark muss cannot be separated from the dinamics that breaks electroweak symmetry.
Sorry for the typos
I forgot to mention that when the mass above 650 GeV could not be possible in the golden decay channel:H.--.> ZZ .---.>l +l -l +l -. And the cross section is too low to observe the four lepton decay mode with. Ie earlier the value (of 650 GeV )was considered too big. And i should write down "the mass of the top quark" (or more precise the top quark mass cannot be separated)
http://prola.aps.org/abstract/PR/v183/i5/p1245_1
http://arxiv.org/PS_cache/arxiv/pdf/0712/0712.0609v2.pdf
http://arxiv.org/PS_cache/arxiv/pdf/0904/0904.0864v2.pdf http://www.sciencedirect.com/science?_ob=ArticleURL&_udi=B6TVN-46YCBPK-51&_user=10&_coverDate=03%2F30%2F1989&_rdoc=1&_fmt=high&_orig=search&_sort=d&_docanchor=&view=c&_acct=C000050221&_version=1&_urlVersion=0&_userid=10&md5=ea6fd62510d32671864887748e8fc5a9
Extended technicolor was introduced by Eichten , Susskind , Lane and Dimopoulos in somewhere in the late seventies of last century . For a quark of mass mq ~ 1 GeV, and with lyamdaTC ~ 250 GeV, one estimates lambdaETC~ 15 TeV. In short it will be possible assumed on the basis of the foregoing, , that, METC will be at least this large.In addition to the ETC proposal for quark and lepton masses, Eichten and Lane have calculated (in time) that the size of the ETC representations required to generate all quark and lepton masses suggests that there will be more than one electroweak doublet of technifermions If so, there will be more (spontaneously broken) chiral symmetries and therefore more Goldstone bosons than are eaten by the Higgs mechanism. These must acquire mass by virtue of the fact that the extra chiral symmetries are also explicitly broken, by the standard-model interactions and the ETC interactions. These "pseudo-Goldstone bosons" are called technipions.ETC interactions generate and the coupling of technipions to quark and lepton pairs, one expects the couplings to be Higgs-like; i.e., roughly proportional to the masses of the quarks and leptons.One can note, that technipions are expected to decay to the heaviest and pairs allowed.
Sorry for that I should write down: "One can note, that technipions are expected to decay to the heaviest quarks (qq_) and lepton pairs (ll_) allowed" instead: "One can note, that technipions are expected to decay to the heaviest quarks (qq_) and lepton pairs (ll_) allowed ". And one can add that: - the minimal technicolor model includes three Nambu-Goldstone bosons that give rise to the longitudinal components of the weak gauge bosons and the color neutral. In the past the typical walking mode featured matter fermions (the technifermions) transforming according to the fundamental representation of the underlying technicolor gauge theory. These models are also known as one Standard Model like family models. But according to the allegations that have recently made Francesco Sannino by computing the precision parameters in an exactly computable region of the phase diagram of strongly interacting theories and comparing it with the phenomenological results for QCD-like dynamics, a large number of flavors gauged under the electroweak symmetry leads to large deviations with respect to electroweak precision measurements. Near walking dynamics is insufficient to reduce the associated S-parameter to phenomenologically acceptable values.
according to the allegations that have recently made by Francesco Sannino
http://ru.arxiv.org/PS_cache/arxiv/pdf/1006/1006.0207v1.pdf Нет я не преследую цель поправлять самого себя , когда я проявляю сильно выраженную рассеяность(я это делать не люблю), просто хочу доводитть начатое до логического конца( пальцы разминаю ).... http://hep.sdu.dk/HighEnergy/Home.html ... А что касается ссылок , то это ну раз я об этом говорил, пусть буду, кому интересно пусть смотрит...
And yet this is not advertising of ideas, I just give myself gamble (or rather I went into the excitement)
это ретроспективно, но интересно
http://arxiv.org/PS_cache/hep-ph/pdf/0202/0202255v1.pdf
Well, let it be heard as follows: Even in complete absence of the Higgs sector in the SM the electroweak symmetry breaks due to the condensation of the quark bilinear in QCD, but this mechanism not be able to explain the contribution of the weak gauge bosons masses. At this time I am ungodly miss all the details. And if the only sourse contributing would be only in QCD, then to the spontaneous breaking of electroweak symmetry one would be have: Mw ~ 29MeV or Mw = gFp / 2, where Fp is the pion decay constant. This contribution (of course) could be sieve very small with respect to the value of the Mw mass that one typically neglects it . According to the idea of ( let it be) of technicolor one augments the SM with another interaction simmilar to QCD
the SM with another interaction simmilar to QCD bottles (of course) with new a new dinamics scale on the order of the electroweak one. It is sufficient that the new gauge theory is asymptotically free and has global symmetry able to contain the SM SU (2) lxUy (1) global symmetries.
Ладно пока всем ;-)))
Well, what else happened:The antimatter was caught in a magnetic trap (in November 2010): At CERN .. An antihydrogen atom is made from a negatively charged antiproton and a positively charged positron, the antimatter counterpart of the electron. The objective - both for ALPHA and for a competing CERN experiment called ATRAP - is to compare the energy levels in antihydrogen with those of hydrogen, to confirm that antimatter particles experience the same electromagnetic forces as matter particles, a key premise of the standard model. For physicists, a bit of antimatter is a quite attractive gift of course. The objective - both for ALPHA and for a competing CERN experiment called ATRAP - is to compare the energy levels in antihydrogen with those of hydrogen, to confirm that antimatter particles experience the same electromagnetic forces as matter particles, a key premise of the standard model. A bit of history will not hurt: - Dirac realised that his relativistic version of the Schredinger wave equation for electrons predicted the possibility of antielectrons. These were discovered by D. Anderson in 1932 and named positrons (a contraction of "positive electrons"). Although Dirac did not himself use the term antimatter, its use follows on naturally enough from antielectrons, antiprotons etc. A complete periodic table of antimatter was envisaged by C. Janet in 1929. One way to denote an antiparticle is by adding a bar over the particle's symbol. The baryon asymmetry problem in physics refers to the apparent fact that there is an imbalance in baryonic matter and antibaryonic matter in the universe. Neither the standard model of particle physics, nor the theory of general relativity provide an obvious explanation for why this should be so; and it is a natural assumption that the universe be neutral with all conserved charges. Almost all matter observable from the Earth seems to be made of matter rather than antimatter. Many scientists believe that this preponderance of matter over antimatter (known as baryon asymmetry) is the result of an imbalance in the production of matter and antimatter particles in the early universe, in a process called baryogenesis. If antimatter-dominated regions of space existed, the gamma rays produced in annihilation reactions along the boundary between matter and antimatter regions would be detectable. The amount of matter presently observable in the universe only requires an imbalance in the early universe on the order of one extra matter particle per billion matter-antimatter particle pairs. Antiparticles are created everywhere in the universe where high-energy particle collisions take place. High-energy cosmic rays impacting Earth's atmosphere (or any other matter in the solar system) produce minute quantities of antiparticles in the resulting particle jets, which are immediately annihilated by contact with nearby matter. Recent observations by the ESA. 'S INTEGRAL satellite may explain the origin of a giant cloud of antimatter surrounding the galactic center. Antimatter may exist in relatively large amounts in far away galaxies due to cosmic inflation in the primordial time of the universe. NASA is trying to determine if this is true by looking for X-ray and gamma-ray signatures of annihilation events in colliding superclusters. Well, one consideration assumes that the antiparticles are also produced in any environment with a sufficiently high temperature (mean particle energy greater than the pair production threshold). Well, one consideration assumes that the antiparticles are also produced in any environment with a sufficiently high temperature (mean particle energy greater than the pair production threshold). During the period of baryogenesis, when the universe was extremely hot and dense, matter and antimatter were continually produced and annihilated. The presence of remaining matter, and absence of detectable remaining antimatter also called baryon asymmetry, is attributed to violation of the CP-symmetry relating matter and antimatter. The exact mechanism of this violation during baryogenesis remains a mystery.Positrons can also be produced by radioactive beta ^ () decay, but this mechanism can occur both naturally and artificially. Antihydrogen is the antimatter counterpart of hydrogen. Whereas the common hydrogen atom is composed of an electron and proton, the antihydrogen atom is made up of a positron and antiproton. The standard symbol for antihydrogen is H_. According to the CPT theorem of particle physics, antihydrogen atoms should have many of the characteristics regular hydrogen atoms have, ie they should have the same mass, magnetic moment, and transition frequencies between its atomic quantum states. For example, excited antihydrogen atoms are expected to glow with the same color as that of regular hydrogen ... The first antihydrogen was produced by a team of researchers under the lead of Walter Oelert at the CERN laboratory in 1995. The experiment took place in the LEAR, where antiprotons, which were produced in a particle accelerator, were shot at xenon clusters. When an antiproton gets close to a xenon nucleus, an electron-positron-pair can be produced, and with some probability the positron will be captured by the antiproton to form antihydrogen. The probability for producing antihydrogen from one antiproton was only about 10 ^ (-19), so this method is not well suited for the production of substantial amounts of antihydrogen. The experiments done at CERN were later, (somewhere at the end of the last century), reproduced at Fermilab where a somewhat different cross section for the process was identified. Both experiment resulted in highly energetic, or warm, antihydrogen, which were unsuitable for detailed study. Subsequently CERN build the Antiproton Decelerator in order to support efforts towards creating low energy antihydrogen which could be used for tests of fundamental symmetries. Production and detection of cold antihydrogen atoms also happened at CERN in 2002, but those were short-lived yu (Although they made antimatter they couldn't store it, because the anti-atoms touched the ordinary-matter walls of the experiments within millionths of a second after forming and were instantly annihilated - completely destroyed by conversion to energy and other particles.). But in this year, antihydrogen was obtained again, but "lasted" a little longer. Antiparticles behave predictably in the presence of electric or magnetic fields and so can be contained in a special magnetic container called a Penning trap. Antihydrogen, which has no net electric charge, is much harder to contain. Production begins by creating antiprotons, which are slowed down by the Antiproton Decelerator at CERN, then stored in the magnetic Penning trap and further cooled. Positrons are supplied by a radioactive sodium-22 source and then cooled as well. These two antiparticles are then allowed to mix and, if this is done at low enough energies, they combine to form antihydrogen. At this point, there is a problem: the antihydrogen is chargeless and can no longer be contained in the magnetic Penning trap. The researchers constructed what they called an ALPHA apparatus, which features a novel superconducting magnetic trap that interacts with the antihydrogen's magnetic moment. The magnetic moment of an individual atom comes from the structure or interaction of the orbiting particle (the positron) and the nucleus (the antiproton) and allows the particle to interact with electric fields in a weak manner. The ALPHA trap can confine antihydrogen in the ground state if it's kept at temperatures of less than half a Kelvin. One challenge of this experiment is mixing the antiprotons and positrons at relatively low velocities such that antihydrogen can form efficiently. Hydrogen has been an experimentaly important tool in the development of QM and QED as the most successful. A number of reasons should be expected that antihydrogen will play an equally important role in the developed of modern physics. Firstly, using the same experimental methods as developed for hydrogen spectroscopy, high precision comparison of the two systems may be achieved. Since such comparative measurements do not require knowlege of the absolute frequency the need for ultra-precise frequency standarts is alleviated, thus paving the way towards yet higher accuracies. Secondly, since antihydrogen is a mostly electromagnetic system (weak interaction, or parity violating, effects are small and the same for hydrogen and antihydrogen) the interpretation of any deviation between hydrogen and antihydrogen is less model dependent than in case of the kaon mass difference. High-resolution laser spectroscopy of antihydrogen could open a new field for very stringent tests of the fundamental CPT theorem. (As already mentioned). . Well, what's next. Laser cooling and laser spectroscopy techniques are also essential for possible measurements of the gravitational force on antihydrogen. Two collaborations have formed with the goal of precision measurements on magnetically trapped antihydrogen using the new Antiproton Decelerator (AD) at CERN. The rate for spontaneous radiative recombination of antiprotons and positrons is small since photon emission is a slow process on the time scale of a collision. ALPHA stored atoms of antihydrogen, consisting of a single negatively charged antiproton orbited by a single positively charged anti-electron (positron). While the number of trapped anti-atoms is far too small to fuel the Starship Enterprise's matter-antimatter reactor, this advance brings closer the day when scientists will be able to make precision tests of the fundamental symmetries of nature. Measurements of anti-atoms may reveal how the physics of antimatter differs from that of the ordinary matter that dominates the world today.Now in principle, the simplest process is spontaneous radiative recombination (SRR): e ^ () p_ .--.> H_ hnu. The rate for this process can be increased by laser simulation: e ^ () p_ khnu .--.> H_ (k 1) hnu. A different approach to enhance the rate of anihydrogen formation is based on three-body recombination (TBR): e ^ () e ^ () p_ .--.> H_ e ^ (-). The above reactions require that two plasmas charge (antiprotons and positrons) are trapped and brought into constant. Alternatively recombination by crossing a beam of positronium (either in the ground state or in low-lying exited state) with antiprotons has been proposed: Ps p_ .--.> H_ e ^ (-) and Ps * p_ .--.> H_ * e ^ (-).
Анонимные комментарии не принимаются.
Войти | Зарегистрироваться | Войти через:
Комментарии от анонимных пользователей не принимаются
Войти | Зарегистрироваться | Войти через: